Anatomy and Physiology: You've Got Some Nerve!
You've Got Some Nerve!
Sorry, I couldn't resist the bad joke at the expense of the spinal cord. One of the great fields of medical research is neurology, and the various subdisciplines, such as neuroanatomy. We can thank a Mr. Phineas Gage, whose tragic railway accident in Vermont, in which a bar was explosively propelled through his skull on September 13, 1848, forever altering his personality and temperament, for the acceleration of our knowledge of the brain. Although cases such as these, and strokes mentioned above, are very valuable, as is much of the imaging technology, there are some very real barriers to research.
We can learn a great deal by studying animal brains, but how do you ask them their feelings, or to solve a mathematical problem? We can ask that of people, but how do you measure microscopic anatomical changes without harming the patient? Certain ethical issues are insurmountable. Apart from this, how do you look at a brain in action, at the microscopic level, without altering it? It is a little like Schrdinger's famous cat and the quantum theory of superposition. Yet, despite these limitations, a great deal has been learned about the brain and the spinal cord.
Meninges
To start off, how do you protect your brain, given its consistency, somewhat akin to soft cheese? If you remember the cranial bones, that's great, but they are awfully hard on the inside, too, so you need a bit of protection from banging into the inner wall. As a matter of fact, that is what a concussion is, in which your head hits a surface, and your brain, through inertia, keeps moving until it bangs into the inside of the skull. There is no way to stop that with a major impact, but you can lessen it, and even prevent it with weaker impacts, through a series of structures called the cranial meninges.
The meninges, which are also found around the spinal cord, where they are called spinal meninges, are composed of multiple layers, which serve not only to protect the CNS by cushioning it (the way a water bed would stop you from hitting the floor), but also to help supply nutrients via the cerebrospinal fluid (CSF), which will be discussed later in this section (see Figure 20.1). Imagine that you are practicing an ancient medical technique called trepanation, in which you cut a hole in your skull, presumably to release the “evil spirits” within. Anyway, what would you see as you began to cut?
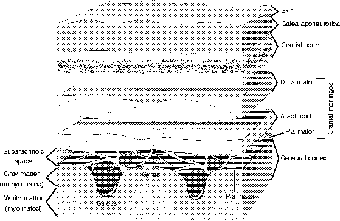
Figure 20.1These are the many layers of tissue connecting the brain to the skull. (LifeART©1989-2001, Lippincott Williams & Wilkins)
First of all, you have to cut your way through the skin, and then, depending on where you cut, you have to cut through either muscle or connective tissue to get to the periosteum, and then the cranial bone. This is where your saw comes in handy. My anatomy students are always a bit taken aback by how hard it is! Luckily, brain surgeons today have miniature radial saws. After cutting through the bone, things get interesting. If you expect to see the hills and valleys (gyri and sulci) right away, think again.
The first layer you'll see will be the outer dura mater, which forms a continuous outer layer for both the brain and the spinal cord. There are two layers for the dura mater, given these hard-to-remember names: the outer layer, and the inner layer. Around the spinal cord is an extra space above the dura mater, between it and the walls of the vertebral foramen; it has a familiar name that will suddenly make sense: the epidural layer (of childbirth fame). Between the two layers there may be a dural sinus (you will meet them by name later) filled with CSF.
Just inside the inner layer of the dura mater is the arachnoid layer, which occasionally has villi extending into the dura mater. Under the dura, between the dura mater and the arachnoid, is the subdural space; it is speculated that this is just an artifact of tissue preparation, and doesn't exist in a living person. Does the name arachnoid make you think of spiders? The pattern of collagen and elastic fibers under the arachnoid layer, in the unromantically named subarachnoid space, look enough like a spider's web to warrant the name. CSF also flows through the subarachnoid space. At the bottom of this layer is the pia mater, which is immediately surrounding the cerebral cortex of the brain (cortex = the outer layer).
Shades of Gray
If you cut a brain from front to back with a deli slicer (not that I'm suggesting you do so), you will see clear layers in gray and white. The outer layer, the cerebral cortex, is gray, as are layers closer to the thalamus and hypothalamus, but much of the rest of it is white. As you learned in The Nervous System, the gray layer consists of unmyelinated nerve tissue, such as cell bodies, dendrites, and unmyelinated axons. This area is likely to have numerous synapses, and it is at these synapses that the real work of the brain is done. For example, processing of sensory input, and the coordination of muscle responses happen in this gray area (see the section on sensory and motor control later in this section).
The white matter, with all the oligodendrocytes (see The Nervous System) covering the axons with myelin, is basically just transmitting information (via nerve impulses), either to areas within the same hemisphere, or in areas from one hemisphere to another. Upon dissection it is actually possible to remove a great deal of the other tissue in the brain, so as to highlight the actual tracts of nerve fibers in the brain. One of the better-known areas is the corpus callosum, which carries the impulses between the left and right hemispheres. For this reason, the corpus callosum is made of white matter.
The spinal cord also has gray and white sections (see Figure 20.2). The gray sections, with all their synapses, make a great deal more sense in the context of the sensory and motor divisions of the spinal nerves. The white matter, however, carrying impulses up and down, is covered here.
If you look at the image showing various cross-sections of the spinal cord, you will begin to get a sense of some of the sensory (to the brain) and motor (from the brain) pathways. The concepts of sensory and motor are explored in more detail later in this section. In the sensory pathway, to begin with, there are a number of neurons involved. A stimulus first triggers a receptor, which may or may not be part of a neuron; in any case, that triggers a first-order neuron in the PNS, which will ultimately trigger a second-order neuron in the gray matter of the CNS.
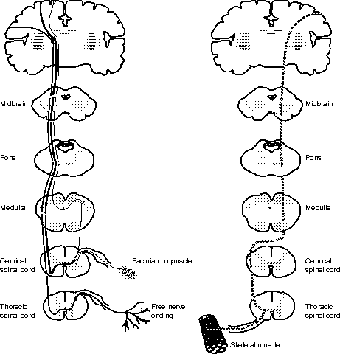
Figure 20.2This figure shows various transverse sections of the spinal cord and brainstem, as well as the sensory and motor pathways. (LifeART©1989-2001, Lippincott Williams & Wilkins)
The Big Picture
Writing a section like this is a bit of a false construct. Think about it. This section is basically about the CNS (brain and spinal cord), and the next section is about the nerves of the PNS. In reality they are inextricably linked. The spinal nerves also connect the CNS with every part of the body via sensory and motor nerves. If you take any one body system, or even part of a system, out of the picture, things start to unravel!
The axon from that neuron continues up the spinal cord (in the white matter) and crosses over to the opposite side in the pyramids of the medulla oblongata, the area immediately superior to, and continuous with, the spinal cord. The second-order neuron triggers a third-order neuron in the thalamus (the base of the brain), which in turn triggers a neuron in the primary sensory cortex, which enables you to consciously perceive the stimulus that started this relay race in the first place.
The odd butterfly shape in the center of the spinal cord is made of the anterior and posterior gray horns. Surrounding them, however, are regions of white matter. These tracts of myelinated axons form four basic areas: the posterior column, the anterior column, and the left and right lateral columns. Don't forget that all sensory and motor paths in the spinal column ultimately cross over from the right to the left side, and vice versa. Where this crossover occurs has a great deal to do with the origin of the impulse.
Flex Your Muscles
Once again, don't forget to break down the words. Take anterior spinothalamic tract as an example. A tract is a pathway for neural impulses (which will be white matter, since there are no synapses). Where does it go? As the name says, from the spine to the thalamus. Where is it in the spinal cord? Easy! The front! In the middle of the front, or slightly off to one side? Well, since we are bilateral, and this is only for one side of the body, it must be slightly off to the side!
The impulses from receptors for delicate sensation, such as vibration, pressure, and gentle touch, travel up the posterior column pathways. Crude touch and pressure (as opposed to fine touch) travel up the anterior spinothalmic tract in the anterior column. Sensation of pain and temperature is carried in the lateral spinothalmic tract in the lateral column. The fine sensations cross over in the medulla, but the latter two (crude touch and pain) cross over in the gray matter at the level of the initial spinal nerve, and then proceed straight up.
Proprioception, the perception of muscle position (see The Senses), travels in two separate pathways due to different destinations. The posterior column pathway takes it, along with all the other spinal sensory information, to the primary sensory cortex in the parietal lobe (see the sections on sensory and motor control, and on brain lobes, in this section). As you shall see in The Senses, the special senses (smell, vision, taste, hearing, and balance) all have specific cranial nerves associated with them, and do not all go to the parietal lobe.
The other pathway for proprioception goes to the cerebellum, which coordinates complex muscle movements (see the following section), via the posterior spinocerebellar tract, with the message crossing over in the gray matter at the location of the spinal nerve. What is interesting here is that sensory information that crosses over also goes up on the same side as it entered. This means that each side of the cerebellum receives impulses from both sides of the body. A nifty way to ensure that our movements are balanced when they need to be!
As far as muscle control goes, in addition to the cerebellum, the primary motor cortex in the frontal lobe controls muscle contraction. (Here, and elsewhere, some of the information is covered in more detail later in this section.) The impulses from the frontal lobe travel through large pathways called the cerebral peduncle, which is in the front of the mesencephalon and ultimately cross over mostly in the pyramids (a section of crossed pathways—left to right and vice versa) of the medulla oblongata. The neurons that cross over go along the lateral corticospinal tract (frontal cortex to the spine), but there are other neurons that don't cross over until the level of the spinal nerve. Those neurons send their impulses down the anterior corticospinal tract.

Excerpted from The Complete Idiot's Guide to Anatomy and Physiology © 2004 by Michael J. Vieira Lazaroff. All rights reserved including the right of reproduction in whole or in part in any form. Used by arrangement with Alpha Books, a member of Penguin Group (USA) Inc.
To order this book direct from the publisher, visit the Penguin USA website or call 1-800-253-6476. You can also purchase this book at Amazon.com and Barnes & Noble.